Electrochemistry
- Electrolytic cells & Galvanic cells
- The function of Salt Bridge
- Redox reaction, EMF of the cell, standard electrode potential
- Standard hydrogen electrode (SHE)
- Nernst equation and its application to Chemical cell
- Equilibrium Constant from Nernst Equation
- Electrochemical Cell and Gibbs Energy
Electrochemistry: It is a branch of chemistry that deals with the relationship between chemical energy and electrical energy and their interconversions.
Redox Reactions: Oxidation is the process that involves the loss of electrons & reduction is a process in which it involves the gain of electrons. The reactions which involve both that reaction simultaneously are called a redox reaction.
Electrochemical Cells: These are devices that convert the chemical energy of some redox reactions to electrical energy. They are also called Galvanic cells or Voltaic cells. An example for a Galvanic cell is Daniel cell.
It is constructed by dipping a Zn rod in ZnSO4 solution and a Cu rod in CuSO4 solution. The two solutions are connected externally by a metallic wire through a voltmeter and a switch and internally by a salt bridge.
A salt bridge is a U-tube containing an inert electrolyte like NaNO3 or KNO3 in a gelly like substance. The functions of a salt bridge are:
- To complete the electrical circuit
- To maintain the electrical neutrality in the two half cells.
- Cu2+ + 2 e– → Cu(s) (reduction half reaction)
- Zn(s) → Zn2+ + 2 e– (oxidation half reaction)
These reactions occur in two different portions of the Daniel cell. The reduction half-reaction occurs on the copper electrode while the oxidation half-reaction occurs on the zinc electrode. These two portions of the cell are also called half-cells or redox couples. The copper electrode may be called the reduction half-cell and the zinc electrode, the oxidation half-cell.
Electrode Potential: This tendency of a metal to lose or gain an electron when it is in contact with its own solution is called electrode potential.
Standard electrode potential (E0): The electrode potential measured at standard conditions.ie at 298K,1 atm pressure, and at 1 molar concentration.
Standard hydrogen electrode (SHE): The reference electrode used to measure single electrode potential. Its potential is assumed to be zero. It consists of a platinum wire dipped in HCl of 1 molar concentration. Hydrogen gas at 1 atm. is passed through the solution. The electrode can be represented as Pt, H2 /H+(1M).
Nernst Equation For A Cell Reaction:-
Let us consider a general equation
aA + bB → cC + dD
E = E0cell – 2.303 RT × log [C]c [D]d
nF [A]a [B]b
Where E0 is the standard electrode potential,
R is the gas constant (8.314 JK–1 mol–1)
F is Faraday constant (96500 C mol–1)
T is the temperature in Kelvin.
Nernst equation can be written as:
Putting the above values Nernst equations can be written as
Equilibrium Constant from Nernst Equation:
Electrochemical Cell and Gibbs Energy of the Reaction:
Electrical work done in one second is equal to electrical potential multiplied by total charge passed. The reversible work done by a galvanic cell is equal to a decrease in its Gibbs energy and therefore if the emf of the cell is E and nF is the amount of charge passed and ∆G is the Gibbs energy of the reaction, then
∆G = – nFEcell
If the concentration of all the reacting species is unity, then Ecell = E0cell and we have
∆G0 = – nFE0 cell
Thus, from the measurement of E0cell, we can calculate the standard Gibbs energy of the reaction.
Conductivity Molar conductivity and Electrolysis
Resistance (R): The electrical resistance is a hindrance to the flow of electrons. Its unit is the ohm (Ω). The resistance of a conductor is directly proportional to the length of the conductor (Ɩ) and inversely proportional to the area of cross-section (A) of the conductor.
R α Ɩ /A
R = ρ Ɩ /A
where ρ (rho) is a constant called resistivity or specific resistance. Its unit is ohm-meter (Ω m) or ohm-centimeter (Ω cm).
Resistivity (ρ): It defined as the resistance offered by a conductor having unit length and unit area of cross-section.
Conductance (G): It is the inverse of resistance.
i.e. G = 1/R. Its unit is ohm-1 or mho or Siemens (S)
Measurement of the conductivity of ionic solutions:
We know that conductivity G = ƙ × A/ Ɩ
So conductivity, ƙ = G × Ɩ/A
The quantity Ɩ/A is called cell constant (G*). It depends on the distance between the electrodes and their area of cross-section. Its unit is m-1.
i.e. Conductivity = Conductance × Cell Constant
Molar conductivity (λm):
It is the conductivity of 1 mole of an electrolytic solution kept between two electrodes with a unit area of cross-section and at a distance of unit length. It is related to the conductivity of the solution by the equation,
λm= ƙ/C (where C is the concentration of the solution)
Or, λm = 1000 ƙ/M (where M is the molarity)
The unit of molar conductivity is Ω-1 cm2 mol-1 or S cm2 mol-1.
Variation of conductivity and Molar conductivity with concentration:
Both conductivity and molar conductivity change with the concentration of the electrolyte. For both strong and weak electrolytes, conductivity always decreases with dilution. This is because conductivity is the conductance of the unit volume of the electrolytic solution. As dilution increases, the number of ions per unit volume decreases, and hence the conductivity decreases.
For strong electrolytes, as dilution increases, the force of attraction between the ions decreases and hence the ionic mobility increases. So molar conductivity increases. When dilution reaches maximum or concentration approaches zero, the molar conductivity becomes maximum and it is called the limiting molar conductivity (λ0m).
The variation of λm for strong and weak electrolytes is shown in the following graphs:
For strong electrolytes, the value of λ0m can be determined by the extrapolation of the graph. But for weak electrolytes, it is not possible since the graph is not a straight line. So their λ0m values are calculated by applying Kohlrausch’s law of independent migration of ions.
Electrochemistry Class 12 Notes
Kohlrausch’s law: Molar conductance at infinite dilution of a strong electrolyte is equal to the sum of molar ionic conductance of the cation and anion at infinite dilution.
eg: λ0m NaOH = λ0m Na+ + λ0m OH-
Applications of Kohlrausch’s law:
(i) Determination of λ0m of weak electrolytes:
By knowing the λ0m values of strong electrolytes, we can calculate λ0m of weak electrolytes. For e.g. we can determine the λ0m of acetic acid (CH3COOH) by knowing the λ0m of CH3COONa, NaCl, and HCl as follows:
λ0m (CH3COONa) = λ0CH3COO– + λ0Na+ …………. (1)
λ0m (HCl) = λ0H+ + λ0 Cl- …………….. (2)
λ0m (NaCl) = λ0Na+ + λ0Cl- ………….. (3)
(1) + (2) + (3) gives:
λ0m (CH3COONa) + λ0m (HCl) – λ0m (NaCl) = λ0CH3COO– + λ0Na+ + λ0H+ + λ0Cl– – λ0Na+ – λ0Cl– = λ0CH3COOH
(ii) Determination of degree of dissociation of weak electrolytes:
By knowing the molar conductivity at a particular concentration (λcm) and limiting molar conductivity (λ0m), we can calculate the degree of dissociation (α) as,
By using α, we can calculate the dissociation constant of acid as:
Electrolytic Cells and Electrolysis: In an electrolytic cell, the electrical energy is converted to chemical energy. The dissociation of an electrolyte by the passage of electricity is called electrolysis. For e.g.
When CuSO4 solution is electrolyzed by Cu electrodes, Cu is deposited at the cathode and Cu2+ ions are liberated from the anode.
Quantitative Aspects of electrolysis – Faraday’s laws
(1) Faraday’s first law:
The amount of substance(m) deposited at the electrode during electrolysis is directly proportional to the quantity of electricity(q) passed through the electrolyte.
m α Q
OR
m = ZQ
Where Z is a constant called electrochemical equivalent. Z = equivalent weight/96500
But the quantity of electricity is the product of current in ampere (I) and time in second (t).
i.e. Q = It
Therefore, m= Zit
(2) Faraday’s Second Law:
When the same amount of electricity is passed through different electrolytic solutions, the amount of Substance deposited is proportional to the chemical equivalent weights.
For e.g. when the same quantity of electricity is passed through solutions of two substances A and B, then
Mass of A deposited = Equivalent mass of A
Mass of B deposited Equivalent mass of B
Products of electrolysis: The products of electrolysis depend on the nature of the electrolyte and the type of electrodes used. If the electrode is inert (e.g. Pt, gold, etc.), it does not participate in the electrode reaction. While if the electrode is reactive, it also participates in the electrode reaction.
For e.g. if molten NaCl is electrolyzed, Na is deposited at the cathode, and chlorine is liberated at the anode. NaCl → Na+ + Cl–
At cathode: Na+ + e– → Na
At anode: Cl– → ½ Cl2 + e–
Primary Secondary Batteries and Corrosion
Batteries: A battery is basically a galvanic cell in which the chemical energy of a redox reaction is converted to electrical energy. They are of mainly 2 types – primary batteries and secondary batteries.
Primary batteries: Here the reaction occurs only once and after use over a period of time, they become dead and cannot be reused. E.g. Dry cell, mercury button cell, etc. (Primary cells cannot be recharged and reused).
(i) Dry Cell (ii) Mercury Cell
Secondary cells: A secondary cell can be recharged and reused again and again. Here the cell reaction can be reversed by passing a current through it in the opposite direction. The most important secondary cell is the lead storage cell, which is used in automobiles and investors.
Lead – Storage Battery
Fuel Cells: These are galvanic cells that convert the energy of the combustion of fuels like hydrogen, methane, methanol, etc. directly into electrical energy.
One example of a fuel cell is the Hydrogen – Oxygen fuel cell, which is used in the Apollo space programme. Here hydrogen and oxygen are bubbled through porous carbon electrodes into a concentrated aqueous sodium hydroxide solution. To increase the rate of electrode reactions, catalysts like finely divided platinum or palladium metal are filled into the electrodes.
Corrosion: It is a process of eating away of metals on their surfaces, it is an unwanted process as it results in a loss of mass of metals. In this process, metal surface reacts with atmospheric oxygen to form a layer of oxide. It is an electrochemical reaction.
The most familiar example of corrosion is the rusting of iron. It occurs in presence of water and air. It is a redox reaction. At a particular spot of the metal, oxidation takes place and that spot behaves as an anode. Here Fe is oxidized to Fe2+. 2 Fe (s)→2 Fe2+ + 4 e–
Electrons released at the anodic spot move through the metal and go to another spot on the metal and reduce oxygen in presence of H+. This spot behaves as a cathode. The reaction taking place at this spot is:
The overall reaction is: 2Fe(s)+O2(g) + 4H+(aq) → 2Fe2 +(aq)+ 2 H2O (l )
The ferrous ions (Fe2+) are further oxidized to ferric ions (Fe3+) and finally to hydrated ferric oxide (Fe2O3. x H2O), which is called rust.
Methods to prevent corrosion:
(i) By coating the metal surface with paint, varnish, etc.
(ii) By coating the metal surface with another electropositive metal like zinc, magnesium, etc. The coating of metal with zinc is called galvanization and the resulting iron is called galvanized iron.
(iii) By coating with an anti-rust solution.
(iv) An electrochemical method is to provide a sacrificial electrode of another metal (like Mg, Zn, etc.) which corrodes itself but saves the object (sacrificial protection)
1. Electrochemistry is the branch of chemistry which deals with the relationship between electrical energy and chemical energy and inter-conversion of one form into another.
2. An electrochemical cell consists of two metallic electrodes dipped in electrolytic solutions. The cells are of two types:
(a) Electrolytic cells (b) Galvanic cells
3. A galvanic cell consists of two half cells. Each half cell contains an electrolytic solution and a metallic electrode. The electrode at which- oxidation takes place is called an anode and the electrode at which reduction takes place is called the cathode. The half-cells are separated from each other by means of a porous pot or a salt bridge.
4. The passage of current from one electrode to the other indicates the existence of potential difference between them. This difference of potential which causes current to flow from the electrode of higher negative potential is called the electromotive force (emf).
5. Electrical energy = Emf (volts) x Quantity of electricity (coulombs)
6. The potential of SHE is assigned an arbitrary value of zero. E° = 0 V. It is used as a reference electrode for measuring the standard electrode potentials. .
7. When the elements are arranged in order of their standard electrode potentials, a series known as electrochemical series is obtained.
8. Standard emf of a cell,
E0cell =E0cathode – E0node = E0Riglit – E0Left
9. ΔG° = -nFE0cell
If E0cell is positive, ΔG° would be negative and reaction would be spontaneous. If E0cell is
negative, ΔG° would be positive and the reaction would be non-spontaneous.
10. A species with higher standard reduction potential has greater tendency to accept electrons to undergo reduction or vice versa.
11. The potential of an electrode in contact with its ions in solution varies with the concentration of the ion. Thus, for a redox reaction,
12. For an electrochemical cell for which the overall reaction is
13. The equilibrium constant, Aofcell can be relate to standard emf of cell
14. Resistance is the measure of obstruction to the flow of current.
where S = specific resistance or resistivity. Unit of R is ohm.
15. Resistivity is defined as the resistance of a conductor of 1cm length and having an area of cross-section equal to 1cm2.
unit of resistivity is ohm-cm
16. Conductance is reciprocal of resistance, i. e.,
Unit of conductance is ohm-1 or mho or Siemen(S).
17. Specific conductance Λsp is reciprocal of specific resistance.
Specific conductance is thus defined as the conductance of a solution taken in a cell whose electrodes are at unit distance apart from each other and each having an area equal to 1 cm2. Unit of specific conductance is ohm-1 cm-1 or S cm-1.
18. Molar conductance (Λm) is defined as the conductance of the volume of solution which contains one mole of the solute and is placed between two parallel electrodes which are one centimetre apart and having sufficient area to hold the whole of the solution.
Unit of molar conductance is Ω-1 cm2 mol-1 or S cm2 mol-1
19.
where C = concentration of solution in moles per litre (or Molarity).
20. The electrical conductance through metals decreases with increase in temperature.
21. The electrolytic conductance increases with increase of temperature.
22. Effect of Dilution on
(а) Equivalent conductance: The value of equivalent conductance increase with dilution and attains a maximum value at infinite dilution.
(b) Specific conductance: The value of specific conductance decreases with dilution as the number of current carrying particles i.e., ions present per cm3 of solution decreases on dilution.
(c) Molar conductance: The value of molar conductance increases with dilution and finally attains a maximum value at infinite dilution.
23. Variation of molar conductance with concentration:
(a) Strong electrolytes:
(b) Weak electrolytes:
24. According to Kohlrausch law of independent migration of ions, the limiting molar conductivity of an electrolyte can be expressed as the sum of the contributions of the cation and the anion of the electrolyte.
where v+, v– are the number of cat ions and anions per unit formula of the electrolyte respectively; λ0+, and λ0– are the limiting molar conductivities of the cation and anion respectively.
25. Faraday’s laws of electrolysis:
(a) First law: The amount of a substance deposited or liberated at an electrode is directly proportional to the quantity of electricity passed through the electrolyte. Mathematically,
where m = mass of substance deposited or liberated.
Mass of substance A deposited or liberated Mass of substance B deposited or liberated current in amperes t = time in seconds, and Z = constant called electrochemical equivalent.
(b) Second law: When the same quantity of electricity is passed through solutions of different electrolytes, the weight of different substances deposited or liberated at the respective electrodes are proportional to their chemical equivalent weights.
26.
27. The charge on one mole of electrons is approximately equal to 96500 coulombs. This quantity of electricity is called Faraday constant (F).
28. A battery consists of two or more galvanic cells connected in series. There are two kinds of batteries:
(a) Primary batteries: In primary batteries, when the reactants have been converted into
products, no more electricity is produced. The cell reaction cannot be reversed and the battery becomes dead.
(b) Secondary batteries: In secondary batteries (or cells), the cell reaction can be reversed by passing electricity through the battery (charging). It means that the battery can be used again and again through a large number of discharging and charging cycles.
29. The most common example of secondary battery is the lead storage battery.
30. Electrical cells that are discharged to convert the energy from the combustion of fuels (hydrogen, carbon monoxide, methane, etc.) directly into the electrical energy are called fuel cells.
31. The corrosion of metals is an electrochemical process. It occurs in presence of water and oxygen.
Electrochemistry is that branch of chemistry which deals with the study of production of electricity from energy released during spontaneous chemical reactions and the use of electrical energy to bring about non-spontaneous chemical transformations.
Importance of Electrochemistry
- Production of metals like Na, Mg. Ca and Al.
- Electroplating.
- Purification of metals.
- Batteries and cells used in various instruments.
Conductors
Substances that allow electric current to pass through them are known as conductors.
Metallic Conductors or Electronic Conductors
Substances which allow the electric current to pass through them by the movement of electrons are called metallic conductors, e.g.. metals.
Electrolytic Conductors or Electrolytes
Substances which allow the passage of electricity through their fused state or aqueous solution and undergo chemical decomposition are called electrolytic conductors, e.g., aqueous solution of acids. bases and salts.
Electrolytes are of two types:
- Strong electrolytes The electrolytes that completely dissociate or ionise into ions are called strong electrolytes. e.g., HCl, NaOH, K2SO4
- Weak electrolytes The electrolytes that dissociate partially (ex < 1) are called weak electrolytes, e.g., CH3COOH, H2CO3, NH4OHH2S, etc.
Electrochemical Cell and Electrolytic

A galvanic cell is an electrochemical cell in which spontaneous redox processes occur which allow the continuous flow of electrons through the conductor, while redox reactions are induced by an external source of current in an electrolytic cell. The mechanical energy is transformed to electrical energy in a galvanic cell, whereas the electrical energy is converted to chemical energy in an electrolyte cell. Here, in the article, let us learn more differences between galvanic and electrolytic cell. Given below in a tabular column, the difference between electrolytic cells and Galvanic cells is listed neatly.
Galvanic Cells vs Electrolytic Cells
Galvanic Cells | Electrolytic Cells |
Spontaneous redox reactions convert the chemical energy to an electric energy | Non-spontaneous redox reactions convert the electric energy to a chemical energy |
Electric energy is generated by redox reactions | Electric energy brings about the chemical reaction with the help of an external source |
The cathode is the positive electrode and anode is the negative electrode | The anode is the positive electrode and cathode is the negative electrode |
The process of oxidation takes place at the anode and the reduction process occurs at the cathode | Here, the oxidation process occurs at the anode while the reduction process takes place at the cathode |
Half cells are set up in different containers and are connected through salt bridges | Electrodes are kept in the same container in a molten or solution electrolyte |
Application lies in Batteries | Application lies in purifying copper and electroplating |
From the above differences between galvanic cells and electrolytic cells, we can conclude that a galvanic cell produces electric current with the help of the chemical reactions that occur spontaneously in it, while an electrolytic cell does the opposite, that is, it brings about chemical reactions with the help of an electric current from an external source.
A cell of almost constant emf is called standard cell. The most common is Weston standard cell.
Galvanic cell is also called voltaic cell.
General Representation of an Electrochemical Cell
Other features of the electrochemical cell are
There is no evolution of heat.
The solution remains neutral on both sides.
The reaction and now of electrons stops after sometime.
Daniell Cell
An electrochemical cell of zinc and copper metals is known as Daniell cell. It is represented as

By convention cathode is represented on the RHS and anode on the LHS.
Function of salt bridge
- It completes the circuit and allows the flow of current.
- It maintains the electrical neutrality on both sides. Salt-bridge generally contains solution of strong electrolyte such as KNO3, KCL etc. KCI is preferred because the transport numbers of K+ and Cl– are almost same.
Transport number or Transference number The current flowing through an electrolytic solution is carried by the ions. The fraction of the current carried by an ion is called its transport number or transference number. Thus.
Transport number of cation. nc = (current carried by cation/total current)
Transport number of cation. na = (current carried by anion/total current)
Evidently nc + na = 1
Electrode Potential
When an electrode is in contact with the solution of its ions in a half-cell, it has a tendency to lose or gain electrons which is known as electrode potential. It is expressed in volts. It is an intensive property, i.e., independent of the amount of species in the reaction.
Oxidation potential The tendency to lose electrons in the above case is known as oxidation potential. Oxidation potential of a half-cell is inversely proportional to the concentration of ions in the solution.
Reduction potential The tendency to gain electrons in the above case is known as reduction potential. According to IUPAC convention, the reduction potential alone be called as the electrode potential unless it is specifically mentioned.
E°red = – E°oxidalion
It is not possible to determine the absolute value of electrode potential. For this a reference electrode [NHE or SHE] is required. The electrode potential is only the difference of potentials between two electrodes that we can measure by combining them to give a complete cell.
Standard electrode potential The potential difference developed between metal electrode and solution of ions of unit molarity (1M) at 1 atm pressure and 25°C (298 K) is called standard electrode potential.
It is denoted by E°.
Reference Electrode
The electrode of known potential is called reference electrode. It may be primary reference electrode like hydrogen electrode or secondary reference electrode like calomel electrode.
Standard hydrogen electrode (SHE) Standard hydrogen electrode (SHE). also known as normal hydrogen electrode (NHE), consists of platinum wire, carrying platinum foil coated with finely divided platinum black. The wire is sealed into a glass tube. placed in beaker containing 1 M HCl. The hydrogen gas at 1 atm pressure is bubbled through the solution at 298K. Half-cell is pt H2 (1 atm) H+ (1 M)

In SHE. at the surface of plantinum, either of (he following reaction can take place
2H+(ag) + 2e– → H2G Reduction
H2(g) → 2H+(ag) + 2e– Oxidation
The electrode potential of SHE has been fixed as zero at all temperatures.
Its main drawbacks are
- It is difficult to maintain 1 atm pressure of H2 gas.
- It is difficult to maintain H+ ion concentration 1 M.
- The platinum electrode is easily poisoned by traces of impurities.
Hence, calomel electrodes are conveniently used as reference electrodes, It consists of mercury in contact with Hg2 Cl2 (calomel) paste in a solution of KCl.
Electromotive Force (emf) of a Cell
It is the difference between the electrode potentials of two half-cells and cause flow of current from electrode at higher potential to electrode at lower potential. It is also the measure of free energy change. Standard emf of a cell,


Electrochemical Series
It is the arrangement of electrodes in the increasing order of their standard reduction potentials.
Standard Electrode Potential at 298 K


Appications of Electrochemical Series (ECS)
1. The lower the value of E°, the greater the tendency to form cation.
M → Mn+ + ne–
Metals placed below hydrogen in ECS replace hydrogen from di1 acids but metals placed above hydrogen cannot replace hydrogen from dil acids.
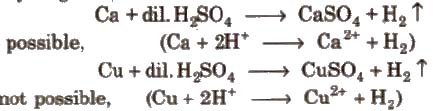
3. Oxides of metals placed below hydrogen are not reduced by H2 but oxides of iron and metals placed above iron are reduced by H2·
- SnO, PbO, CuO are reduced by H2
- CaO, K2O are not reduced by H2·
4. Reducing character increases down the series.
5. Reactivity increases down the series.
6. Determination of emf; emf is the difference of reduction potentials of two half-cells.
- Eemf = ERHS – ELHS
If the value of emf is positive. then reaction take place spontaneously, otherwise not.
7. Greater the reduction potential of a substance, oxidising power. (e.g.. F2 > Cl2 > Br2 > I2)
8. A negative value of standard reduction potential shows that it is the site of oxidation.
9. Oxides of metals having E°red ≥ 0.79 will be decomposed by heating to form O2 and metal.
HgO (s) → Hg(l)(1/2)O2(g)
(E°Hg2+/Hg = 0.79V)
Nernst Equation
The relationship between the concentration of ions and electrode potential is given by Nernst equation.
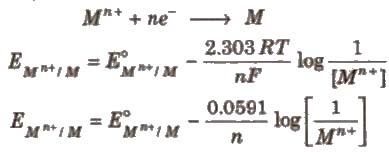
For a electrochemical cell,

Concentration of pure solids and liquids is taken as unity.
Nernst equation and Kc
At equilibrium
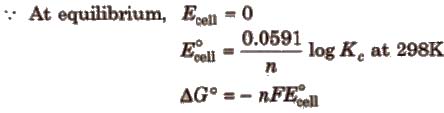
Here, ΔG° is the standard Gibbs free energy change.
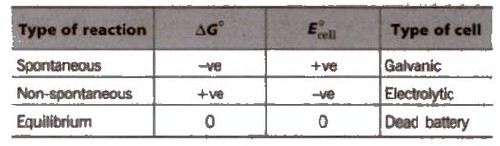
Relationship between free energy change and equilibrium constant
ΔG° = – 2.303RT log Kc
Concentration Cells
(i) Electrode concentration cells Two hydrogen electrodes or different pressures are dipped In the same solution of electrolyte,
e.g..
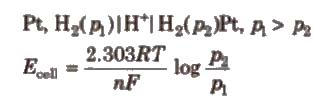
(ii) Electrolyte concentration cells Electrodes are the same but electrolyte solutions have different concentrations, e.g..
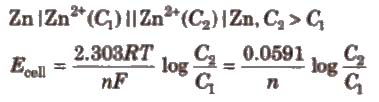
Conductance (G)
It is the ease of flow of electric current through the conductor. It is reciprocal of resistance (R).
G = (1/R), units ohm-1 mhos or Ω-1
Specific Conductivity (K)
It is the reciprocal of specific resistance.
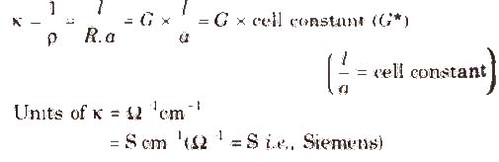
Unit of cell constant is cm-1 or m-1.
Specific conductivity decreases on dilution. This is because concentration of ions per cc decreases upon dilution.
Molar Conductivity (Λm)
The conductivity of all the ions produced when 1 mole of an electrolyte is dissolved in V mL of solution is known as molar conductivity.
It is related to specific conductance as
Λm = (k x 1000/M)
where. M = molarity.
It units are Ω-1 cm2 mol-1 or S cm2 mol-1.
Equivalent conductivity (Λm)
The conducting power of all the ions produced when 1 g-equivalent of an electrolyte is dissolved in V mL of solution, is called equivalent conductivity. It is related to specific conductance as
Λm = (k x 1000/N)
where. N = normality.
Its units are ohm-1 cm2 (equiv-1) or mho cm2 (equiv-1) or S cm2 (g-equiv-1).
Debye-Huckel Onsagar equation It gives a relation between molar conductivity, Λm at a particular concentration and molar conductivity Λm at infinite dilution.
Λm = Λ0m – √C
where, b is a constant. It depends upon the nature of solvent and temperature.
Factors Affecting Conductivity
(i) Nature of electrolyte The strong electrolytes like KNO3 KCl. NaOH. etc. are completely ionised in aqueous solution and have high values of conductivity (molar as well as equivalent).
The weak electrolytes are ionised to a lesser extent in aqueous solution and have lower values of conductivity (molar as well as equivalent) .
ii) Concentration of the solution The concentrated solutions of strong electrolytes have Significant interionic attractions. which reduce the speed of ions and lower the value of Λm. and Λeq.
The dilution decreases such attractions and increase the value of Λm and Λeq.

The limiting value, Λ0m or Λ∞m. (the molar conductivity at zero concentration (or at infinite dilution) can be obtained extrapolating the graph.
In case of weak electrolytes, the degree of ionisation increases dilution which increases the value of Λm and Λeq. The limiting value Λ0m cannot be obtained by extrapolating the graph. ~
limiting value, Λ0m, for weak electrolytes is obtained by Kohlrausch law.
(iii) Temperature The increase of temperature decreases inter-ionic attractions and increases kinetic energy of ions and their speed. Thus, Λm and Λeq increase with temperature.
Kohlrausch Law
At infinite dilution, the molar conductivity of an electrolyte is the sum of the ionic conductivities of the cations and anions, e.g., for AxBy.
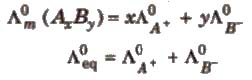
Applications
(i) Determination of equivalent/molar conductivities of weak electrolytes at infinite dilution, e.g.,

(ii) Determination of degree of dissociation (α) of an electrolyte at a given dilution.

The dissociation constant (K) of the weak electrolyte at concentration C of the solution can be calculated by using the formula
kc = (Cα2/1 – α)
where, α is the degree of dissociation of the electrolyte.
(iii) Salts like BaSO4 .., PbSO4‘ AgCl, AgBr and AgI which do not dissolve to a large extent in water are called sparingly soluble salts.
The solubility of a sparingly soluble salt can be calculated as
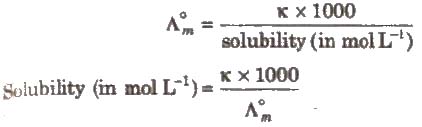
Electrolysis
It is the process of decomposition of an electrolyte when electric current is passed through either its aqueous solution or molten state,
- In electrolytic cell both oxidation and reduction takes place in the same cell.
- Anode is positively charged and cathode is negatively charged, In electrolytic cell.
- During electrolysis of molten electrolyte, cations are liberated at cathode. while anions at the anode.
- When two or more ions compete at the electrodes. the ion with higher reduction potential gets liberated at the cathode while the ion with lower reduction potential at the anode.
For metals to be deposited on the cathode during electrolysis, the voltage required is almost the same as the standard
electrode potential. However for liberation of gases, some extra voltage is required than the theoretical value of the standard electrode potential. The extra voltage thus required is called over voltage or bubble voltage.
How to Predict the Products of Electrolysis?
When an aqueous solution of an electrolyte is electrolysed, if the cation has higher reduction potential than water (-0.83 V), cation is liberated at the cathode (e.g.. in the electrolysis of copper and silver salts) otherwise H2 gas is liberated due to reduction of water (e.g., in the electrolysis of K, Na, Ca salts, etc.) Similarly if anion has higher oxidation potential than water (- 1.23 V), anion is liberated (e.g., Br–), otherwise O2 gas is liberated due to oxidation of water (e.g., in case of F–, aqueous solution of Na2SO4 as oxidation potential of SO2-4 is – 0.2 V).
Discharge potential is defined as the minimum potential that must be applied across the electrodes to bring about the electrolysis and subsequent discharge of the ion on the electrode.
Faraday’s Laws of Electrolysis
1. First law
The amount of the substance deposited or liberated at cathode directly proportional to the quantity of electricity passed through electrolyte.
W ∝ I x t = I x t x Z = Q x Z
- I current in amp, t = time in sec,
- Q = quantity of charge (coulomb)
- Z is a constant known as electrochemical equivalent.
When I = 1 amp, t = 1 sec then Q = 1 coulomb, then w = Z.
Thus, electrochemical equivalent I” the amount of the substance deposited or liberated by passing 1A current for 1 sec (i.e.. 1 coulomb, I x t = Q)
2. Second law
When the same quantity of electricity is passed through different electrolytes. the amounts of the substance deposited or liberated at the electrodes arc directly proportional to their equivalent weights, Thus,
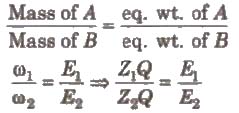
Hence, electrochemical equivalent ∝ equivalent weight.
Batteries
These are source of electrical energy which may have one or more cells connected in series. For a good quality battery it should be reasonably light. compact and its voltage should not vary appreciably during its use.
Primary Batteries
In the primary batteries. the reaction occurs only once and after use over a period of time battery becomes dead and cannot be reused again.
(i) Dry cell or Leclanehe cell
Anode-Zinc container
Cathode-Graphite rod surrounded by MnO2 powder
Electrolyte-Paste of NH4Cl + ZnCl2
Cathode reaction,
2MnO2(s) + 2 NH+4(aq) + 2e– → Mn2O3(s) + 2NH3(g) + H2O(l)
Anode reaction,
Zn(s) → Zn2+(aq) + 2e–
Cell potential 1.25 V to 1.5 V
(ii) Mercury cell
Anode-Zn-Hg amalgam
Cathode-Paste of (HgO + C)
Electrolyte-Moist paste of KOH-ZnO

Secondary Batteries
These cells can be recharged and can be used again and again, e.g.,
(i) Lead Storage battery
Anode-Spongy lead
Cathode-Grid of lead packed with PbO2
Electrolyte-38% H2SO4 by mass
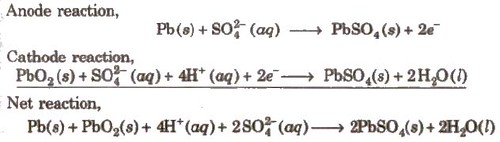
When recharged the cell reactions are reversed.
(ii) Nickel-cadmium storage cell
Anode-Cadmium
Cathode-Metal grid containing NiO2
Electrolyte-KOH solution
Anode reaction,
Cd(s) + 2OH–(aq) → Cd(OH)2(s) + 2e–
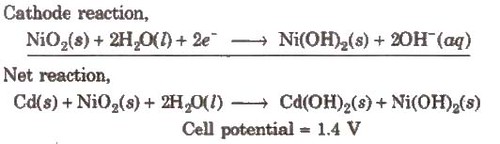
Fuel Cells
Galvanic cells which use energy of combustion of fuels like H2, CH4, CH3OH, etc., as the source to produce electrical energy are called fuel cells. The fuel cells are pollution free and have high efficiency.
Hydrogen-Oxygen Fuel Cell
Electrodes-Made of porous graphite impregnated with catalyst (Pt, Ag or a metal oxide).
Electrolyte-Aqueous solution of KOH or NaOH
Oxygen and hydrogen are continuously fed into the cell.
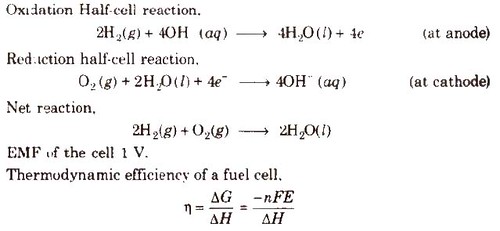
Corrosion
Slow formation of undesirable compounds such as oxides, sulphides or carbonates at the surface of metals by reaction with moisture and other atmospheric gases is known as corrosion.
Factors Affecting Corrosion
- Reactivity of metals
- Presence of moisture and atmospheric gases like CO2, SO2, etc.
- Presence of impurities
- Strains in the metal
- Presence of electrolyte
Rusting of Iron-Electrochemical Theory
An electrochemical cell, also known as corrosion cell, is developed at the surface of iron.
Anode- Pure iron
Cathode-Impure surface
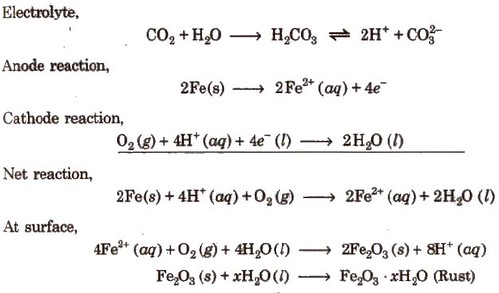
Rusting of iron can be prevented by the following methods :
- Barrier protection through coating of paints or electroplating.
- Through galvanisation or coating of surface with tin metal.
- By the use of antirust solutions (bis phenol).
- By cathodic protection in which a metal is protected from corrosion by connecting it to another metal that is more easily oxidised.
What is the function of a galvanic cell?
The electrochemical cell type is a galvanic cell. It is used to supply electrical current through a redox reaction to the transfer of electrons. A galvanic cell is an example of how to use simple reactions between a few elements to harness energy.
How do you make a voltaic cell?
The basic cell or voltaic cell is made up of two electrodes, one of copper and the other of zinc dipped in a glass vessel solution of dilute sulfuric acid. The current flows from copper to zinc outside the cell and from zinc to copper inside the cell when the two electrodes are connected externally with a piece of wire.
Why cathode is positive in the galvanic cell?
The anode is the electrode where oxidation (loss of electrons) occurs; it is the negative electrode in a galvanic cell since electrons are left on the electrode when oxidation occurs. Therefore, the cathode is a neutral electrode; because there are reduced positive ions of metal atoms.
Is Daniel’s cell a galvanic cell?
Sometimes known as a voltaic cell or Daniell cell is a galvanic cell. One example of a galvanic cell is the common household battery. The electrons flow from one chemical reaction to another occurs through an external circuit that results in the current.
How do you represent a galvanic cell?
A basic voltaic cell is created by immersing in a diluted solution of sulfuric acid a zinc plate and a copper plate. A voltaic cell is an electrochemical cell that produces electrical energy using a chemical reaction. The reactions to oxidation and reduction are divided into half-cell compartments.
An electrochemical cell is a device capable of either generating electrical energy from chemical reactions or using electrical energy to cause chemical reactions.
Table of Content
- What are Electrochemical Cells?
- Half Cells and Cell Potential
- Types of Electrochemical Cells
- Applications of Electrochemical Cells
What is an Electrochemical Cell?
An electrochemical cell is a device that can generate electrical energy from the chemical reactions occurring in it, or use the electrical energy supplied to it to facilitate chemical reactions in it. These devices are capable of converting chemical energy into electrical energy, or vice versa. A common example of an electrochemical cell is a standard 1.5-volt cell which is used to power many electrical appliances such as TV remotes and clocks.
Such cells capable of generating an electric current from the chemical reactions occurring in them care called Galvanic cells or Voltaic cells. Alternatively, the cells which cause chemical reactions to occur in them when an electric current is passed through them are called electrolytic cells.
A diagram detailing the different parts of an electrochemical cell is provided below.
Electrochemical Cell
Electrochemical cells generally consist of a cathode and an anode. The key features of the cathode and the anode are tabulated below.
Cathode Anode Denoted by a positive sign since electrons are consumed here Denoted by a negative sign since electrons are liberated here A reduction reaction occurs in the cathode of an electrochemical cell An oxidation reaction occurs here Electrons move into the cathode Electrons move out of the anode General convention dictates that the cathode must be represented on the right-hand side whereas the anode is represented on the left-hand side while denoting an electrochemical cell.
Half-Cells and Cell Potential
- Electrochemical Cells are made up of two half-cells, each consisting of an electrode which is dipped in an electrolyte. The same electrolyte can be used for both half cells.
- These half cells are connected by a salt bridge which provides the platform for ionic contact between them without allowing them to mix with each other. An example of a salt bridge is a filter paper which is dipped in a potassium nitrate or sodium chloride solution.
- One of the half cells of the electrochemical cell loses electrons due to oxidation and the other gains electrons in a reduction process. It can be noted that an equilibrium reaction occurs in both the half cells, and once the equilibrium is reached, the net voltage becomes 0 and the cell stops producing electricity.
- The tendency of an electrode which is in contact with an electrolyte to lose or gain electrons is described by its electrode potential. The values of these potentials can be used to predict the overall cell potential. Generally, the electrode potentials are measured with the help of the standard hydrogen electrode as a reference electrode (an electrode of known potential).
Primary and Secondary Cells
- Primary cells are basically use-and-throw galvanic cells. The electrochemical reactions that take place in these cells are irreversible in nature. Hence, the reactants are consumed for the generation of electrical energy and the cell stops producing an electric current once the reactants are completely depleted.
- Secondary cells (also known as rechargeable batteries) are electrochemical cells in which the cell has a reversible reaction, i.e. the cell can function as a Galvanic cell as well as an Electrolytic cell.
- Most of the primary batteries (multiple cells connected in series, parallel, or a combination of the two) are considered wasteful and environmentally harmful devices. This is because they require about 50 times the energy they contain in their manufacturing process. They also contain many toxic metals and are considered to be hazardous waste.
Types of Electrochemical Cells
The two primary types of electrochemical cells are
1. Galvanic cells (also known as Voltaic cells)
2. Electrolytic cells
The key differences between Galvanic cells and electrolytic cells are tabulated below.
Galvanic Cell / Voltaic Cell Electrolytic Cell Chemical energy is transformed into electrical energy in these electrochemical cells. Electrical energy is transformed into chemical energy in these cells. The redox reactions that take place in these cells are spontaneous in nature. An input of energy is required for the redox reactions to proceed in these cells, i.e. the reactions are non-spontaneous. In these electrochemical cells, the anode is negatively charged and the cathode is positively charged. These cells feature a positively charged anode and a negatively charged cathode. The electrons originate from the species that undergoes oxidation. Electrons originate from an external source (such as a battery). Applications of Electrochemical Cells
- Electrolytic cells are used in the electrorefining of many non-ferrous metals. They are also used in the electrowinning of these metals.
- The production of high-purity lead, zinc, aluminium, and copper involves the use of electrolytic cells.
- Metallic sodium can be extracted from molten sodium chloride by placing it in an electrolytic cell and passing an electric current through it.
- Many commercially important batteries (such as the lead-acid battery) are made up of Galvanic cells.
- Fuel cells are an important class of electrochemical cells that serve as a source of clean energy in several remote locations.
Frequently Asked Questions on Electrochemical Cells
What is the Function of a Salt Bridge in an Electrochemical Cell?
The salt bridge completes the circuit of an electrochemical cell, thereby allowing the flow of current through it. It also helps maintain the overall electrical neutrality of the cell.
What is Standard Electrode Potential?
The standard electrode potential of an electrode can be defined as the potential difference that arises between the electrode and the electrolyte under standard conditions (Temperature = 298K, pressure = 1 atm, unity concentration of reacting species). It is denoted by the symbol ‘Eocell‘. Click here to learn more about standard electrode potential.
What are the Key Differences between Cathode and Anode?
The cathode of an electrochemical cell is the site at which reduction occurs. It is generally represented by a positive (+) sign. The electrons flow from the anode towards the cathode. In electrochemical cells, the anode is the electrode at which oxidation occurs. It is denoted by a negative (-) sign.
Is it Possible for an Electrochemical Cell to have a Positively Charged Anode or a Negatively Charged Cathode?
Yes, the anode of an electrolytic cell is positively charged (and the cathode is negatively charged). However, oxidation still occurs at the anode despite the negative charge. The chemical reactions that occur in these electrochemical cells are non-spontaneous in nature.
What are electrolytic cells?
Electrolytic cells are a class of electrochemical cells that use electric currents to facilitate the cell reaction. The chemical reaction that occurs inside such cells is commonly referred to as electrolysis. Electrolytic cells can be used to break down bauxite into aluminium and other components. Such cells can also be employed for the electrolysis of water into hydrogen and oxygen.
What is EMF
EMF is abbreviated as the electromotive force which is defined as the maximum potential difference between the two electrodes of a voltaic or galvanic cell.
EMF of a Galvanic cell
What is a galvanic cell
Galvanic cell is one of the most important electrochemical cells. A galvanic cell has a combination of two metal rods known as electrodes. Both the electrodes are immersed in a solution that contains the combination of ions and therefore forms a half cell.
The half cells are connected by a salt bridge and the solution in which both the electrodes are dipped is known as the electrolyte. The chemical reaction happening in this cell is known as a redox reaction. Oxidation takes place in one of the electrodes that acts as an anode and reduction takes place on the other electrode that acts as a cathode.
Cell notation
Some rules need to be taken care while representing an electrochemical cell. The cathode is on the right side and anode on the left side.
The cell is represented by the rule that metals are written first and then the metal ions that are present in the electrolyte. The two needs to be separated by a vertical line. For example Zn | Zn2+.
The molar concentration is written within the brackets as: Zn | Zn2+(1M)
If we take an example of Daniel cell, it can be represented as: Zn | Zn2+|| Cu2+ | Cu
Then cell notation is as follows:
Zn | Zn2+ (1M) || Cu2+ (1M)| CuCell potential or electromotive force (EMF)
It is the potential difference between electrodes in a galvanic cell and is measured in volts.
It can be represented as:
E.m.f of the cell= Half-cell potential at the cathode (right side) – Half-cell potential at the anode (left side)A galvanic cell is an electrochemical cell in which spontaneous redox processes occur which allow the continuous flow of electrons through the conductor, while redox reactions are induced by an external source of current in an electrolytic cell. The mechanical energy is transformed to electrical energy in a galvanic cell, whereas the electrical energy is converted to chemical energy in an electrolyte cell. Here, in the article, let us learn more differences between galvanic and electrolytic cell. Given below in a tabular column, the difference between electrolytic cells and Galvanic cells is listed neatly.
Galvanic Cells vs Electrolytic Cells
Galvanic Cells Electrolytic Cells Spontaneous redox reactions convert the chemical energy to an electric energy Non-spontaneous redox reactions convert the electric energy to a chemical energy Electric energy is generated by redox reactions Electric energy brings about the chemical reaction with the help of an external source The cathode is the positive electrode and anode is the negative electrode The anode is the positive electrode and cathode is the negative electrode The process of oxidation takes place at the anode and the reduction process occurs at the cathode Here, the oxidation process occurs at the anode while the reduction process takes place at the cathode Half cells are set up in different containers and are connected through salt bridges Electrodes are kept in the same container in a molten or solution electrolyte Application lies in Batteries Application lies in purifying copper and electroplating From the above differences between galvanic cells and electrolytic cells, we can conclude that a galvanic cell produces electric current with the help of the chemical reactions that occur spontaneously in it, while an electrolytic cell does the opposite, that is, it brings about chemical reactions with the help of an electric current from an external source.